A complex steel assembly's fate may be determined by the vulnerability of its smallest and least considered components. The bolts or fasteners holding the assembly together are often the areas where corrosion starts first, and where the effects of corrosion may have the most serious consequences.
Although the use of alloy bolts would make a big difference, the vast majority of bolts used in the offshore industry and elsewhere are carbon steel. With some bolts failing in as little as six months, and the design lifetimes of industrial infrastructure being regularly exceeded, a remedy is required that can improve on the often short-lived results achieved using standard coating systems. (See High Pressure Fastener Coating Practices Under Fire: Ian MacMoy Speaks Out for more information.)
This article explores corrosion in bolted systems and how to protect against it.
Bolt Corrosion Mechanisms: Why Do Bolts Rust so Easily?
If we look closely at steel-bolted systems, we find large components assembled and secured using relatively small nuts and bolts. Such assemblies are found on pipelines, machinery and other infrastructures in every corner of the globe. Wherever they are found — in the joints and most particularly in the bolts — is where the greatest corrosion effects are to be found.
Differences in corrosion potential, relative size, mixed materials, damage to coatings during assembly and crevices formed between components are all well-understood factors affecting bolt corrosion, but these are often ignored in practice.
Figure 1 is an example of how the design and construction of an assembly can create unnecessary problems. The photo shows a section of pipework constructed from a combination of low-alloy and high-alloy steels, with a low-alloy steel flange connected to a high-alloy flange using low-alloy bolts and nuts — all supported by pieces of welded angle iron. (Learn about one potential solution in the article Case Study: Large-scale Installation of Encapsulating Technology for Flange Protection.)

Figure 1. Typical problems with mixed metals.
Corrosion in the low-alloy flange is apparent where coating damage has occurred, but most significant is the level of corrosion in the bolts. Here we have two key factors operating:
- Relative size.
- Corrosion potential.
The bolts are of low-alloy steel with a corrosion potential of around 0.85. The flange is high-alloy with a corrosion potential of around 0.5 — a difference of 0.35, well outside acceptable differences. In addition, the bolts and nuts are small relative to the structure to which they are joined, exacerbating the corrosion effect. In some environments, such bolts may show significant corrosion within weeks of installation, as they are affected in the same way as the sacrificial anodes in a cathodic protection (CP) system.
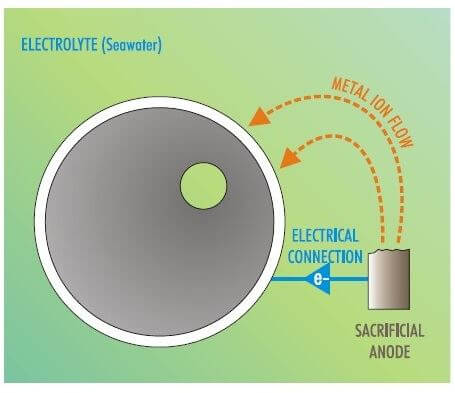

Figure 2. The corrosion process in bolts can cause them to act as sacrificial anodes.
Galvanic Corrosion in Bolts
Galvanic corrosion occurs when two dissimilar metals are in contact with each other while an electrolyte is covering the connecting area. The electro-potential difference between the metals is a driving force for an accelerated corrosion attack to happen. (For more on galvanic corrosion, read: An Introduction to the Galvanic Series: Galvanic Compatibility and Corrosion.)
This process is especially pertinent for bolted systems in seaside, maritime or offshore applications. In these environments, metal structures are often made of aluminum — which, contrary to the common misconception, is extremely corrosive.
In seaside, maritime or offshore applications containing an aluminum structure and metal bolts covered by sodium chloride– (sea salt-) containing water, a galvanic cell may be created. Under these conditions, it is important that bolted joints are carefully designed to minimize the occurrence of galvanic corrosion between the aluminium structure and the metal bolts. Selecting proper materials for these bolted connections may not be easy. In instances where it's not possible to select a material for the bolts that will minimize galvanic corrosion, an insulating layer like a washer can be placed between the two mating areas to avoid contact and thus prevent galvanic corrosion.
(Many people mistakenly believe aluminum is not corrosive because it contains a specific corrosion prevention mechanism involving the formation of a thin protective layer of aluminium oxides, which shields the material from further oxygen access. With that protective layer in place, aluminum's corrosion process normally does not advance. However, under certain circumstances, this process cannot fully protect against corrosion. Galvanic, or bimetallic, corrosion can cause sustained corrosion of aluminium.)
Bolt Damage and Crevice Corrosion
In nuts and bolts, assembly can cause damage and penetration of the coating, which can lead to corrosion. Figure 1 shows a nut after only 18 months in the splash zone, its polytetrafluoroethylene (PTFE) and zinc plating failing within six weeks of exposure.
In a salt-spray test, a zinc/nickel coating would be expected to last at least 1,000 hours before "red rust" occurs. An accelerated weathering test such as this is designed to demonstrate the potential longevity of a coating system on the basis that test conditions are extreme. For example, if it could last 1,000 hours in a salt-spray cabinet, the coating's lifetime should be 10 years under normal conditions.
However, 1,000 hours is only six weeks. In the real world, the bolts shown in Figure 1 have been exposed to constant salt-spray conditions for 18 months, within a structure design life of 20 years.
Crevice corrosion under washers and bolt heads, and disbonded coatings on the threads, also play a role in the litany of problems affecting bolted systems.
In a report on bolt failures as part of a testing program by Det Norske Veritas (DNV), it was shown that washers are more likely to fail than any other component in a bolted system. It is easy to see why this might be true, because washers are the smallest component, trapped in a crevice, subjected to turning forces from both sides, and rarely treated with any care.
What remedies are available? Essentially, three standard remedies exist for corrosion occurring in a bolted assembly:
- Do nothing. Rusting infrastructure around the world is a tribute to the philosophy of putting off until tomorrow that which you should be doing today.
- Take everything apart, clean, recoat, replace and reassemble. Tiis is not really practical if an entire LPG carrier, offshore platform or refinery needs refurbishment every 18 months.
- Clean/blast/recoat the worst affected areas on a routine basis. This is common practice but, once corrosion is in a system, rarely completely successful.
Fastener Corrosion Protection
The best way to protect fasteners from corrosion is to factor its impact into the design process. This is best accomplished by considering both the application for which the fastener will be used as well as the environment in which it will be used, and subsequently ensuring the metals used do not accelerate the corrosion process by reacting against each other.
According to the galvanic theory, materials and products should be placed in such a way as to limit galvanic corrosion occurring. For example, aluminium alloys and copper should not be combined, especially where there is a higher pH due to the environment's salinity. Stainless steel and its alloys would be better paired with copper for high-pH applications, however a protective coating would be necessary to reduce corrosion. Further, it is important to understand that the differences between electrode potentials are affected by the environment in which the application or product is placed.
How to Design Against Corrosion
- Analyze the corrosive environment and its requirements.
- Choose materials with sufficient corrosion resistance and similar galvanic potential.
- Avoid geometric shapes which collect water and dirt, create stress risers and cause erosion.
- Choose a suitable corrosion protection method (e.g., surface coatings, sacrificial anodes, direct currents.)
- Define requirements. For example, determine whether your application should adhere to the ISO 9227 salt spray corrosion test, the ASTM G48 electrochemical corrosion test for stainless steel, theISO 12944 corrosion classes for environments or other such frameworks. (For more on controlling corrosion through design, read: How to Control Corrosion by Improving Design.)
Besides factoring corrosion into the design process, there are several best practices to delay the corrosion of a product or application. These include:
- Choosing materials of a similar electrode potential.
- Using a special paint or coating to create a protective barrier.
- Using sacrificial anodes to protect the core product.
- Introducing a current to offset any galvanic reactions.
While coatings are the standard — and most economic — form of corrosion protection, they are not foolproof. For example, a coating that is corrosion-proofed for a saltwater environment may not be resistant to degreasing solutions.
Finally, it's important to factor in cost to any and all corrosion prevention strategies. If the most effective strategy is out-of-budget, a different approach may be used.
Corrosion Resistant Fasteners
There are a number of materials that have anti-corrosion properties. These include:
Stainless Steel
Stainless steel has corrosion-resistant properties due to the high levels of chromium within the alloy. Stainless steel requires at least 10.5 percent chromium as part of the alloying materials for it to be considered stainless.
The corrosion resistance comes from the chromium, as when exposed to oxygen, the steel forms a layer of chromium oxide. The level of corrosion resistance can be improved by adding molybdenum, titanium, nickel, or copper to the alloy.
Titanium
Titanium has excellent stress corrosion cracking, high corrosion resistance and a number of corrosion-resistant features. (For more on titanium corrosion, read: 5 Things to Know and Understand About Titanium Corrosion.)
Duplex and Super Duplex
Duplex and super duplex stainless steels are used for greater corrosion resistance and resistance to stress corrosion cracking.
Duplex stainless steels are known for their resistance to stress corrosion cracking. Super duplex is the better choice for general corrosion resistance. Super duplex stainless steels are used in a number of demanding applications, offer excellent corrosion resistance and are relied upon heavily in the oil and gas industries.
Inconel
Like titanium, Inconel has a number of grades that all have different properties. Most of these grades are used frequently as fasteners or fixings alongside chemical exposure due to the material's anti-caustic corrosion properties. They also host great resistance to high-purity water corrosion and stress corrosion cracking.
Inconel 600, a nickel-chromium alloy, has very high resistance to a large number of corrosive elements. Inconel 617 is both corrosion- and oxidation-resistant and is often found in chemical processing due to its resistance to a wide range of corrosive liquids.
Incoloy
Incoloy belongs to the same family of superalloys as Inconel, but both have distinct properties. Incoloy has a higher ferrous content than Inconel and is also available in a wide range of grades — all of which are great for corrosion resistant fasteners.
Incoloy 800 contains nickel, chromium and iron and is resistant to high-temperature corrosion, including oxidation and carburizing. Incoloy 825 also contains nickel, chromium and iron but also includes molybdenum, copper and titanium. The addition of the latter materials offers great resistance to stress corrosion cracking, crevice corrosion and pitting. Incoloy 832 resists pitting and hot salt corrosion.
Incoloy 901 has an iron base and contains nickel and chromium, combining high-strength and corrosion resistance at elevated temperatures. Incoloy 925, probably the most common grade for fasteners, imparts excellent resistance to stress corrosion cracking, oxidization, pitting and crevice corrosion.
Hastelloy
Hastelloy is a nickel-molybdenum alloy that, like Inconel and Incoloy, is available in a wide range of grades that are all highly resistant to corrosion. It’s a superalloy, like Incoloy and Inconel, and has high resistance to uniform attack and localized corrosion resistance.
Zirconium
Zirconium fasteners are known for their resistance to some of the harshest environments, including hydrochloric and sulfuric acid. They’re ideal for acidic concentrations but not for ferric or cupric chlorides.
Tantalum
Tantalum fasteners are known for their excellent corrosion resistance within the industry, with all the properties of a metal and similar corrosion resistance to glass.
Anti-Corrosion Coatings for Bolts
Zinc Plating
Fasteners, in particular steel fasteners, are electroplated with zinc for better corrosion resistance. Zinc is the prime material for electroplating fasteners; zinc alloys like zinc-nickel and zinc-iron are also electroplated. Zinc plating involves applying a thin coating of zinc, in microns, to fasteners, giving them a layer of protection from the surrounding environment. The process of electroplating fasteners is called barrel plating.
Zinc-plated fasteners can be subsequently passivated with various colours including clear, yellow and green. This process' corrosion resistance also depends on the subsequent passivation process: Green passivation, for example, has higher corrosion resistance than clear passivation
Hot-Dipped Galvanized Zinc
One of the most effective coatings for steel fasteners in terms of both performance and cost is hot-dipped galvanized zinc. Hot-dip zinc finish is dull and rougher than electroplated zinc coating.
This process is applied to fasteners by dipping the fastener in a bath of molten zinc and then centrifuge spinning the fasteners for even distribution and to remove the excess. A typical coating thickness for hot-dip galvanized zinc is 50 microns (µm) — this is the thickest zinc coating to go onto the fastener, resulting in superior corrosion resistance.
Zinc Flakes
Zinc flake-based coatings that are applied by a dip and spin or spray technique and subsequently cured by heating. The coatings consist predominantly of zinc flakes, usually combined with a small quantity of aluminium. The flakes are about one µm thick and are bonded together with inorganic or organic compounds. These are normally used for high-strength fasteners where hydrogen embrittlement due to zinc plating could be an issue.
These coatings have relatively good coverage and can give good dimensional control and excellent corrosion protection. The corrosion resistance is very high, providing up to 600 hours of salt spray life.
PTFE Coatings
PTFE coatings; fluoropolymers and fluorocarbon-based polymers with multiple strong carbon-fluorine bonds and are characterised by a high resistance to solvents, acids and bases, which provide the material with many key performance strengths.
Rilsan PA 11 Coatings
Rilsan PA 11 coatings may be applied to all types of steel fasteners. Processing is very simple and consists of depositing a Rilsan film onto the fastener's metal surface. Careful surface preparation is necessary for optimum performance. Following this pre-treatment, a primer may be applied to improve the coating's adhesion to the fastener and maximize its anti-corrosion properties. (For more on surface preparation, read: Substrate Surface Preparation for Corrosion Prevention.)
Rilsan has high corrosion resistance in water, wastewater and salt water and has high chemical resistance to hydrocarbons, solvents, acids, salts and alkalis.
Mechanical zinc coatings and phosphating are also used for bolts. Phosphated coatings have lower salt-spray and corrosion resistance.
Corrosion-Inhibiting Sprayable Thermoplastics (CIST)
Corrosion-inhibiting sprayable thermoplastics (CIST) technologies provide a new approach to corrosion control in bolted systems, treating the entire system rather than focusing on any particular part. The system works by excluding corrosion factors such as oxygen and water through an encapsulating barrier coating. It also provides active corrosion protection through the slow release of corrosion-inhibiting oils over the lifetime of the system.
Spray-applied CIST is a zero-VOC (volatile organic compounds) reusable thermoplastic material that can be sprayed onto any substrate size or shape. The system employs a dedicated heating and pumping unit to melt and spray the material, yielding a snug-fitting coating of material that encapsulates the substrate, following every contour. The main functions of the system include the exclusion of electrolyte (water) and oxygen, and the surface coating of inhibiting oil.

Figure 3. Complex structure protected by CIST.
By providing a vector for a film of oil to every surface within the encapsulation, CIST both prevents new corrosion and arrests existing corrosion. Very little surface preparation is required, even on rusty surfaces. Only the removal of loose materials, such as flaking rust and paint, is required.

Figure 4. Main functions of the CIST system.
Field Testing in the North Sea
CIST has been successfully applied since 2003 in the North Sea for protection of prematurely rusting bolts. Severe corrosion was experienced in systems that had been expected to last many years using PTFE-coated, zinc-plated, low-alloy bolts. Bolt replacement was the only obvious remedy. Realizing that this would be both disruptive and expensive, the operators sought alternatives. CIST was selected due to its ability to arrest and prevent corrosion with minimal intervention and surface preparation requirements.

Figure 5. Mixed metal substrate with CIST removed after seven years’ North Sea exposure.
A regular program of removal and inspection was implemented with impressive results such as the example in Figure 5, where a mixed metal substrate is shown to have no corrosion after seven years of exposure (in contrast to the similar substrate illustrated in Figure 1). The seven-year results on inspection included:
- No evidence of new corrosion following encapsulation of previously corroded substrates
- No evidence of galvanic effects or crevice corrosion in protected substrates
- Coating integrity maintained despite heavy contamination
- Inhibiting oil continued to be released after seven years
These operators continue to use CIST across the platform and have not needed to replace any bolts for corrosion failure since the application of CIST.
Field Testing at National Grid Gas Terminal
As a major operator of gas transport pipelines, the UK National Grid needed evidence of CIST performance before recommending its use. Accordingly, in 2005, a series of applications were completed for long-term testing purposes. Removal of CIST and inspections were completed in 2006, 2007, 2009 and finally in 2010 after five years. The five-year results on inspection included:
- No evidence of corrosion was found on any of the CIST-protected substrates
- Inhibiting oil was found to be on every surface within encapsulations
- Although exteriors surfaces were soiled from atmospheric and industrial deposits, the coating remained in good condition with continuing oil deposition on the substrate
Following testing, a preliminary CIST installation on the Bacton interconnector site was completed in 2011, and CIST is now in use on National Grid substations at various sites in the UK, protecting gas-insulated switchgear for the National Grid and other utility companies.
Laboratory Testing
A number of tests have been completed to establish the satisfactory performance of CIST coatings under various conditions, including UV, cryogenic and ignition testing. However, for the purposes of this paper, the focus is on anti-corrosion performance; for this reason, two tests are highlighted here. (For another look at testing, read Lessons Learned from 50 Years of Coatings Testing at SA Water.)
1. Salt Water Deluge Testing: The susceptibility of low-alloy steel assemblies to the corrosion effects of salt water is well documented. In this test, a carbon steel pipe and flange is subject to a constant flow from a 20% saline mixture for five days a week for periods up to six months at 86°F (30°C).

Figure 6. Contrast between uncoated pipe and coated flange.
The flange is protected with CIST, but the pipe remains unprotected. After six months, the constant cycle of salt water and drying has corroded the unprotected pipe. However, under the CIST system, the substrate remains completely clear of corrosion. The saltwater deluge testing results included:
- No ingress of water through the upper seal onto the pipe
- Corrosion occurred on unprotected pipe
- No corrosion within CIST encapsulation
- Coating was unaffected by immersion cycles
2. Hot Salt Fog Testing: An international company considering CIST for wellhead protection subjected a small wellhead section to brutal salt fog testing in a hot salt fog chamber. The substrate was coated with CIST and then large sections of the coating were cut away before the test piece was subjected to a 3,000-hour test in the chamber. The results are shown in Figure 7, with bright steel under the CIST and rust everywhere else.
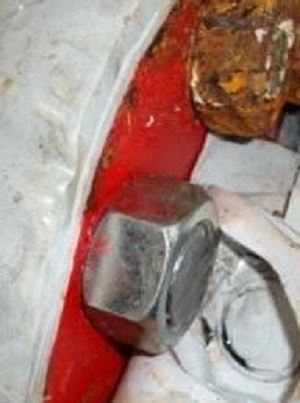
Figure 7. CIST 3,000-hour hot salt fog test.
Independently observed testing by the original developers of CIST showed zero corrosion after more than 10,000 hours in a hot-salt fog chamber—nearly 14 months. Figure 8 shows the control sample and the protected bolt side by side after the testing.

Figure 8. CIST 10,000-hour hot salt fog test.
Results of the developer's hot salt fog tests included:
- Exposed cutaway areas showed severe signs of rust
- CIST-protected areas show no sign of corrosion
- Severe damage to coating did not affect the ability to protect adjacent areas
- Corroded nuts were difficult to turn and remove; impossible to check bolt tension
- Protected nuts rotated freely; bolt tension was unaffected
Traditionally, the application of remedial coatings to corroded bolted steel assemblies required high levels of intervention—disassembly, replacement, blasting and coating—with the expectation that corrosion would reappear relatively quickly.
An effective long-term remedy has an important role to play in infrastructure protection and the reduction of risk to personnel and the environment. Early field use of this new technology shows promise in solving some of these challenges.
- Testing and field applications since 2004 have demonstrated that CIST can provide long-term protection against galvanic corrosion and crevice corrosion.
- CIST provides bolt corrosion control on existing and new-build applications without the need for large-scale intervention.
- CIST corrosion prevention mechanisms provide whole system as well as individual component protection in complex assemblies.
- Non-toxic, reusable and waste-free, CIST helps reduce the environmental impact of corrosion prevention.
Conclusion
In industrial, marine and offshore environments, with equipment life cycles being extended and safety guidelines more stringently applied, corrosion prevention has become increasingly important.
By accounting for corrosion from inception to application, operators will be able to achieve the highest possible corrosion resistance in a bolted system.